Gravity is one of four fundamental forces, alongside electromagnetism and the two types of nuclear force. On very small scales it’s by far the weakest of these forces, but it comes into its own at large distances. That’s because the nuclear forces have a very limited range, while electromagnetic forces tend to get cancelled out because electric charge can be either positive or negative. Gravity, on the other hand, always acts in the same direction – it’s a force of attraction, which means there’s no limit to its range. And it’s a very democratic force, operating with equal effect between any two objects having mass. For these reasons, it’s far and away the most important force governing the universe on large scales.
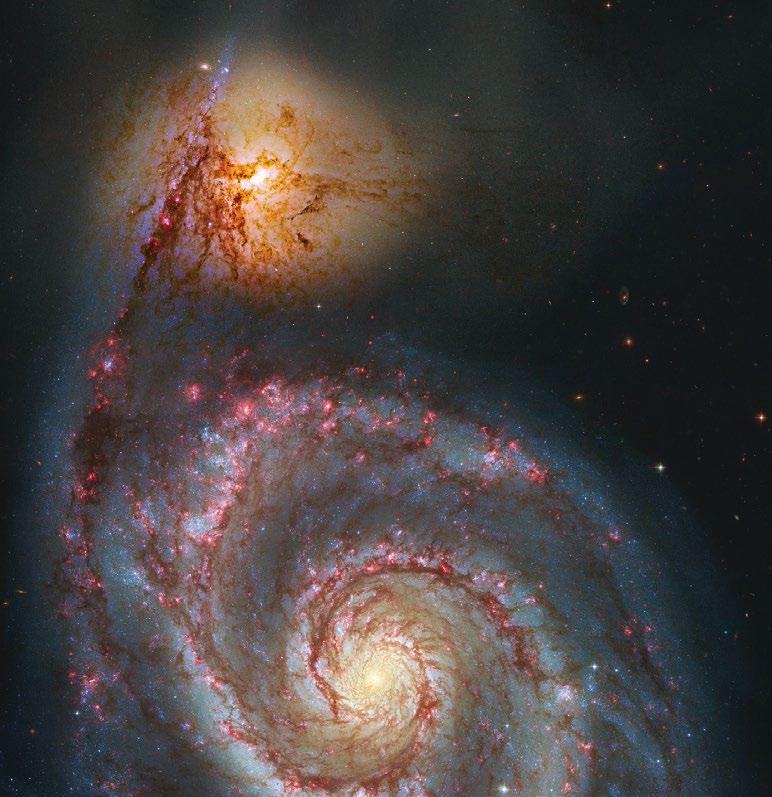
Scientists sometimes speculate what might have happened if the universe was created with a slightly stronger or weaker force of gravity. It turns out that if gravity was a little weaker, the Sun’s core would never have become hot and dense enough to trigger the nuclear reactions that produce sunlight, whereas if it were a little stronger the Sun would have burned out billions of years ago. Needless to say, either of those situations would have been disastrous for any possibility of life on Earth.
Although all objects exert a gravitational pull on all other objects, the only thing that’s close enough and massive enough for us to be aware of it is the Earth itself. The main effect of Earth’s gravity is to give weight to objects. This isn’t the same as the object’s mass, which is simply a measure of the amount of matter it contains, typically expressed in kilograms. An object’s mass remains the same wherever it is in the universe, and determines how strongly it resists any force that’s applied to it. In contrast, an object’s weight is the amount of force that a gravitational field exerts on it. Strictly speaking, weight should be measured in units of force, such as newtons, but because it’s always proportional to mass when we’re on Earth’s surface, we usually measure it in kilograms instead. But on a smaller planet an object’s weight would be lower, even though its mass remains the same. For example, a person weighing 50 kilograms on Earth would only weigh around 18 kilograms on Mars.
Although the strength of Earth’s gravity decreases with increasing distance, it still has a perceptible effect way out in space. It’s what keeps satellites and the Moon in orbit around the planet. In fact, the Moon is large enough for its gravity to have a reciprocal effect on the Earth, which we see in the form of ocean tides.
These are caused by the difference in the strength of the Moon’s gravitational pull on the water on opposite sides of the planet, causing the seas to bulge outwards slightly.
In the wider universe, gravity can produce some spectacular effects. It’s the force that holds galaxies together, and the primary cause of the intricate spiral patterns that many of them display. It’s also responsible for binding multiple galaxies together into large groups and clusters. And gravity is crucial to the formation and behaviour of black holes, too. When a large star runs out of nuclear fuel, it’s because it’s unable to withstand the pull of its own gravity, so it collapses all the way down to a black hole. And the most dreaded effect of all that can befall an object venturing too close to a black hole – spaghettification, or being pulled apart into long, thin strands – happens as a result of the incredibly strong tidal effects of the black hole’s gravitational field.
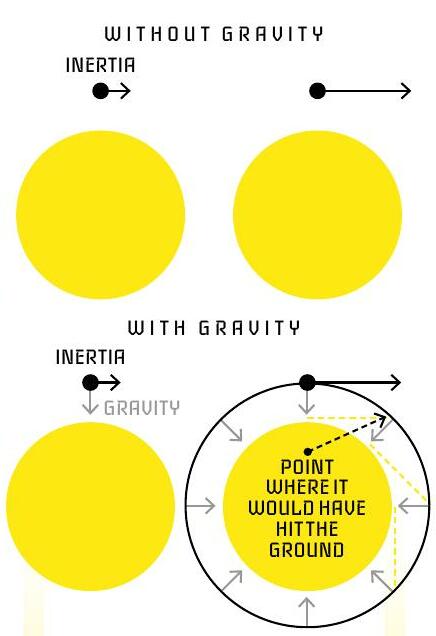
Many people find it confusing that a satellite can stay in orbit without expending any power – particularly when they’re told that this is due to gravity. Surely gravity would just pull the satellite down to Earth? This would be true if it simply remained stationary at an altitude of, say, 200 miles, where gravity is almost as strong as it is down here on the surface of the planet. But the fact is that satellites aren’t stationary – they’re moving at high speed parallel to the surface of Earth. To understand how this works, it helps to consider what would happen in the absence of gravity. Since there’s very little air resistance at that altitude, the satellite would just whiz along in a straight line at constant speed. But because gravity is constantly pulling it towards the centre of Earth, it’s bent round in a big circle instead.
WHO DISCOVERED GRAVITY?
The ancient Greeks believed that gravity was simply a natural tendency of earthly objects to fall towards the ground, whereas celestial objects such as planets obeyed a completely different principle that caused them to move in circles. A proper scientific study of gravity had to wait until the 17th century. Galileo Galilei was the first person to record systematic experiments on the subject, but a few decades later, Isaac Newton went even further. He produced what has become known as his law of universal gravitation, which was a remarkable achievement for two reasons. Firstly, it encapsulated the effects of gravity in the form of a mathematical equation – something that hadn’t been done before for a ‘force of nature’. Secondly, his formula not only explained everyday gravity here on Earth, but the orbits of astronomical bodies such as the Moon and planets as well.
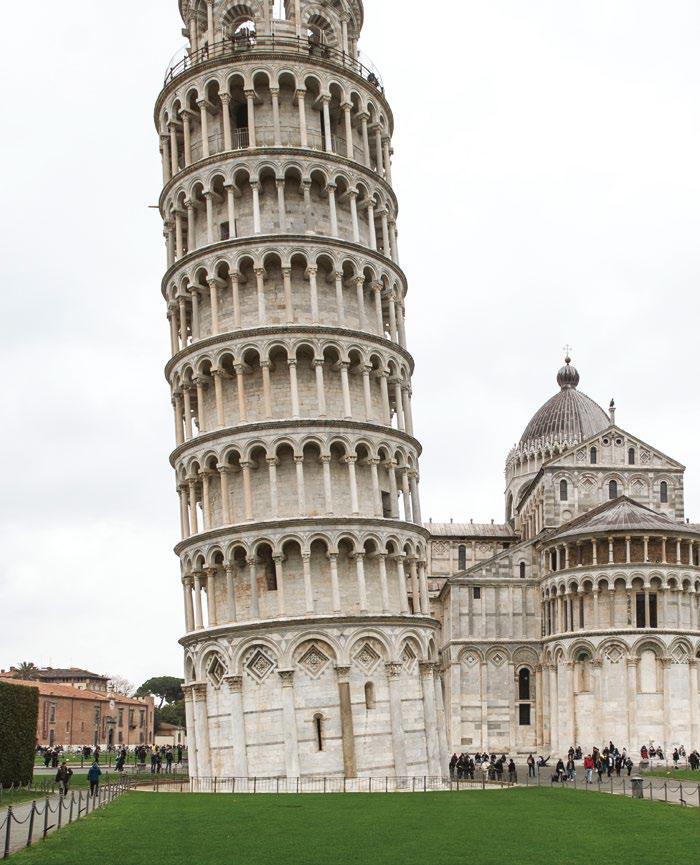
Galileo is reputed to have used the Leaning Tower of Pisa for this dramatic demonstration
1 GALILEO
He climbed to the top of the tower, which is roughly 50 metres above the ground.
2 TWO CANNONBALLS
He simultaneously dropped two cannonballs made of different materials, hence having different weights.
3 THE EFFECT OF GRAVITY
Gravity accelerates all objects at exactly the same rate, regardless of their mass.
4 OUTCOME
The balls hit the ground almost simultaneously, which Galileo expected, unlike most people at the time.
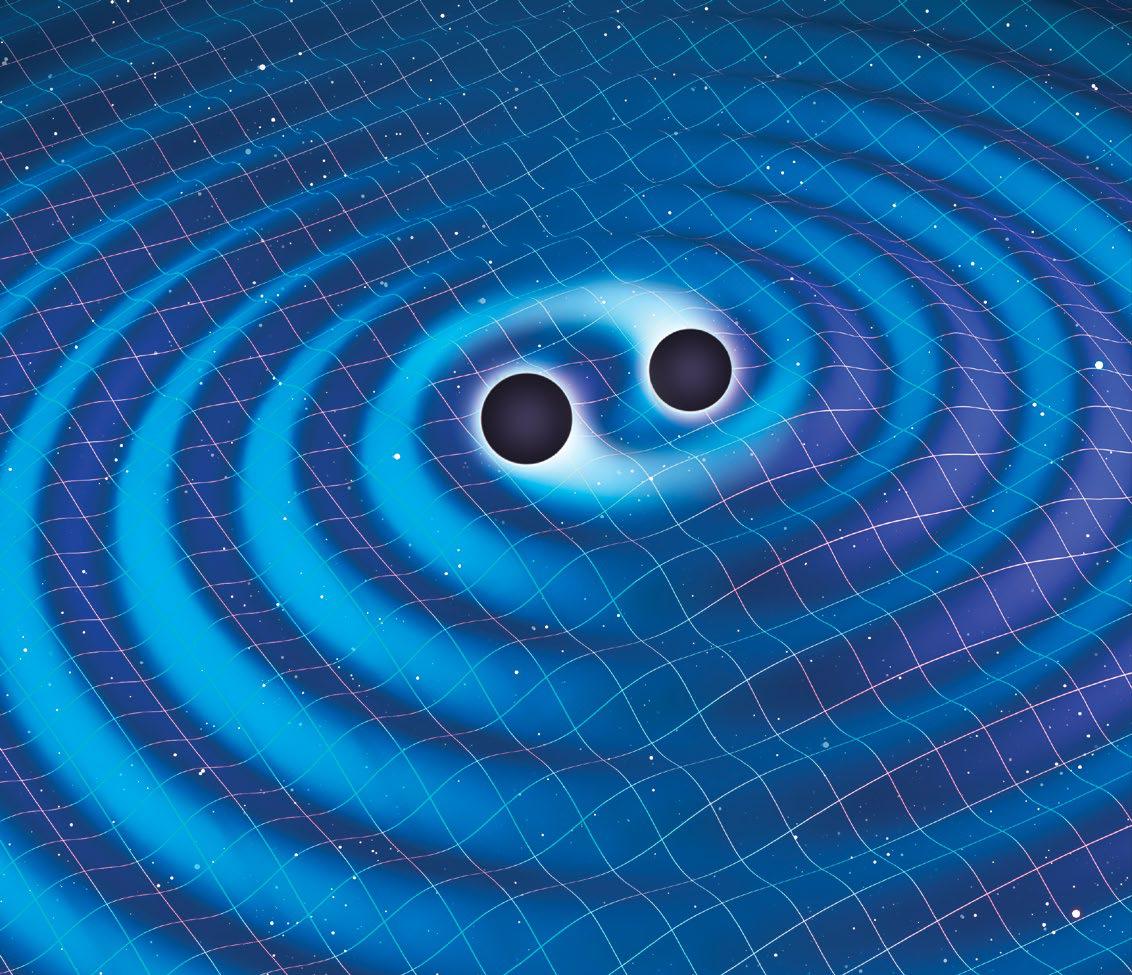
Although Newton’s theory of gravity proved good at explaining the vast majority of astronomical observations, it had a major weakness. In 1905, Einstein produced his theory of special relativity, which says that nothing can travel through space faster than the speed of light. In Newton’s theory, however, gravity is seen as a force between two objects that is transmitted instantaneously over any distance. For this reason, Einstein realised that a new theory of gravity had to be found that was consistent with his universal speed limit.
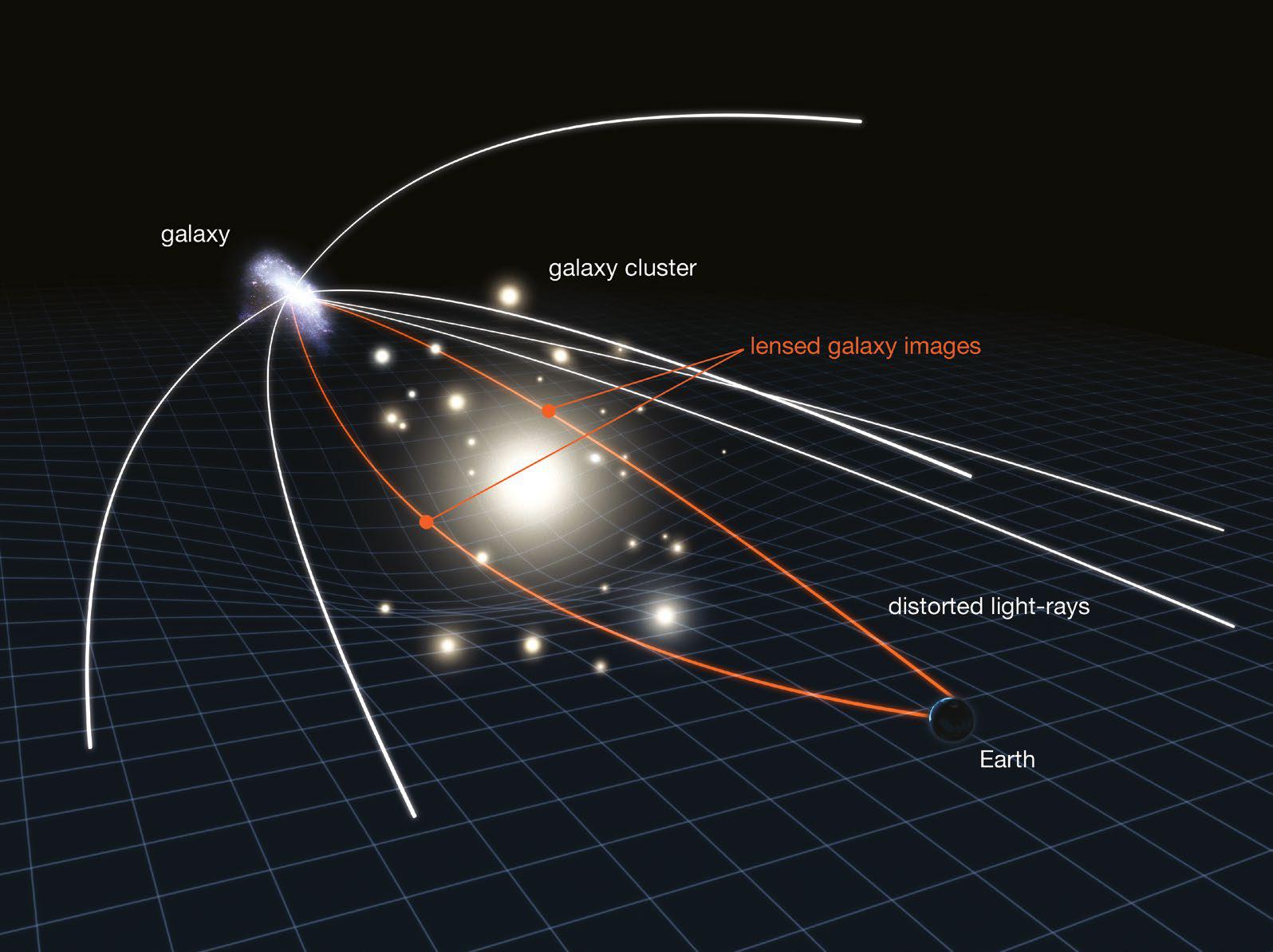
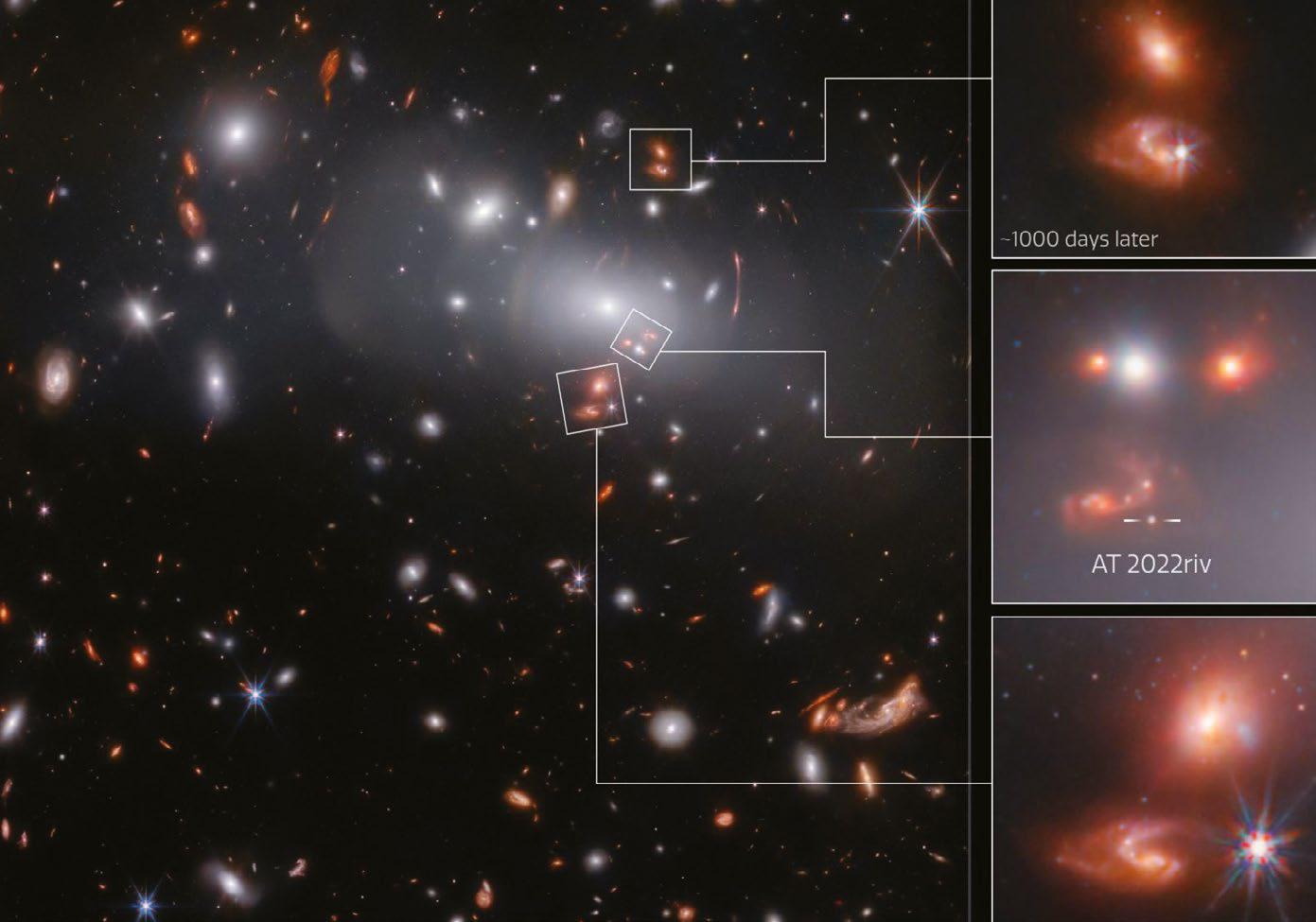